사용자:Khg2202/연습장
![]() |
연대기[편집]
- 과거의 역사는 빅뱅의 연대기을 참조하십시오.
다성 시대[편집]
- 빅뱅 106(백만)년~1014(백조)년 후
우주는 현재 1.38×1010(138억)년 됐다. 지금은 다성 시대다. 빅뱅 1억5500만년 후 첫 별이 생겼다. 그 이후, 별은 수소로 이루어진 크고 차가운 분자 구름의 붕괴에 의해 형성했다. 처음에는 중력 수축에 의해 생성된 에너지에 의한 뜨겁고 밝은 원시성을 생산했다. 잠시 후, 중심부는 수소 핵융합을 하기 충분히 뜨거워졌고 별의 수명이 시작됐다.
질량이 매우 낮은 별은 결국 모든 수소를 배출하고 백색 왜성이 되며, 중간 정도의 질량을 가진 별은 행성상 성운을 만들고 백색 왜성이 되고, 더 무거운 별은 초신성 폭발을 한 후, 중성자별이나 블랙홀이 될 것입니다. 어떤 경우든, 일부 물질들은 성간 물질이 되지만, 중심핵은 성간물질이 되지 못할 것이다. 따라서, 별의 형성에 사용할 수 있는 가스의 공급이 지속적으로 소진되고 있다.
우리 은하와 안드로메다 은하의 충돌[편집]
- 현재로부터 3억년 후(빅뱅 170억년 후)
안드로메다 은하와 우리 은하는 약 250 만 광년 떨어져 초당 약 120km으로 서로를 향해 움직이고 있다. 아마 3억년 후, 우리 은하와 안드로메다 은하는 하나의 은하로 병합 될 수 있다. 안드로메다 은하가 우리 은하를 얼마나 빨리 가로지르는지 알 수 없기 때문에, 충돌이 일어날 것이 확실하지 않다.
은하의 합체[편집]
- 1011(1000억)년~1012(1조)년
은하단에 있는 은하는 1011(1000억)~1012(1조)년 후, 그들의 궤도가 붕괴되고 은하단 전체가 큰 하나의 은하로 병합 될 것으로 예상된다.
국부 초은하단 외부의 은하는 더 이상 감지 불가[편집]
- 2×1012(2조) 년
지금부터 암흑 에너지가 우주를 가속 속도로 계속해서 확장시킨다고 가정하면, 2×1012(2조)년 후, 국부 초은하단밖의 모든 은하는 적색 편이로 인해 감마선조차도 관찰 우주의 크기보다 긴 파장을 방출해야한다. 따라서, 이 은하들은 더 이상 어떤 방식으로든 감지되지 않습니다.
축퇴 시대[편집]
- 1014 (100조) 년~ 1040 (1정) 년
지금으로부터 1014 (100조)년 후에는, 별 생성이 끝나고, 모든 별은 잔해 형태로 남게 된다. 이 시기는 잔해가 붕괴 할 때까지 지속된다.
별 생성 중지[편집]
- 1014 (100조) 년
1014 (100조) 년 혹은 그 이전에 별의 생성은 중지될 것이라 추측된다. 무거운 별은 수소를 빨리 소비한다.(별의 진화 참조). 따라서, 우주에서 가장 오래 살아있는 별은 태양 질량의 0.08배를 가지는 질량이 작은 적색 왜성이며 수명은 1013 (10조) 년이다. 공교롭게도, 이것은 별의 형성이 이루어지는 동안의 시간의 길이와 비교된다. 일단 별 형성이 종료되면 가장 거대한 적색 왜성이 자신의 연료를 소모하고, 핵융합이 중단된다. 적은 질량의 적색 왜성은 식고 백색 왜성이 될 것이다. 행성 이상으로 남아있는 유일한 개체는 갈색 왜성, 백색 왜성, 중성자별, 블랙홀이 될 것이다. 이들의 질량의 대부분은, 약 90 %가 백색 왜성의 형태로 될 것이다. 모든 에너지원의 부재에서 이전에 빛나던 모든 별은 희미하게 될 것이다.
마지막 별이 꺼진 이후 우주는 매우 어둡게 될 것이다. 그럼에도 불구하고, 여전히 우주에서 가끔 빛이 보일 수 있다. 태양 질량의 1.4배의 찬드라세카르 한계 이상으로 충돌한 두 개의 탄소 - 산소 백색 왜성 병합이 일어날 경우가 우주에 불이 켜질 수 있는 방법 중 하나이다. 결과 물체는 Ia형초신성을 생산하고 몇 주 동안 축퇴 시대의 어둠을 밝혀, 폭주 열핵융합을 하게 될 것이다.[1][2] 결합 질량이 찬드라세카르 한계 이상이 아니라 탄소 핵융합 (태양 질량의 약 0.9배)의 최소 질량보다 큰 경우, 탄소 별은 106 (100만) 년 정도의 수명으로, 생성 될 수 있다.[3], p. 91 또한, 최소한 태양 질량의 0.3배의 결합 질량을 가진 두 헬륨 백색 왜성이 충돌하는 경우, 헬륨 별은 몇 억 년의 수명으로, 생성 될 수 있다.[3], p. 91 마지막으로 갈색 왜성이 서로 충돌하는 경우, 1013 (10조) 년을 생존 할 수있는 적색 왜성이 생성 될 수 있다.[1][4]
행성 궤도 붕괴[편집]
- 1015 (1000조) 년
시간이 지남에 따라, 행성의 공전 궤도는 중력파 때문에 붕괴 하거나, 또는 다른 축퇴된 별과 만남에 의한 중력 섭동에 의해 자신의 계에서 방출된다.[5]
별들이 블랙홀에 빨리거나 은하를 탈출함[편집]
- 1019 (1000경) 년~1020 (1해) 년
시간이 지남 과정에서 은하 안의 물체는 맥스웰-볼츠만 분포에 따르는 동적 완화를 한다 [6] 동적 완화는 두 개의 별의 가까운 만남으로 이뤄지지만, 더 빈번한 멀리 떨어진 만남으로도 진행될 수 있습니다.[7] 가까운 만남의 경우, 두 갈색 왜성 또는 축퇴된 별은 서로 가까이 전달합니다. 이 경우, 가까운 만남에 포함 된 물체의 궤적이 약간 변경된다. 많은 만남 이후, 가벼운 물체는 무거운 물체가 운동 에너지을 잃을 때 얻는 경향이 있다.[3], pp. 85–87
동적 완화로 인해 일부 개체는 작고, 조밀한 은하를 뒤에 남겨두고, 은하 탈출 속도에 도달하여 은하를 떠나기에 충분한 에너지를 얻을 것이다. 만남은 조밀한 은하에서 더 자주 있기 때문에, 과정은 가속한다. 결과적으로 대부분의 물체는 은하에서 배출되어 떠나고, 중심부의 초질량 블랙홀에 극히 일부가 (약 1 % ~ 10 %) 떨어진다.[8], §IIIAD;[3], pp. 85–87
핵자 붕괴 시작[편집]
- 1034 년
우주의 다음 진화는 양성자 붕괴의 존재와 속도에 따라 달라집니다. 실험적인 증거는 양성자가 불안정하면, 그것은 적어도 1034 년의 반감기를 가지고 있음을 보여줍니다.[9] 대 통일 이론 중 하나가 올바른 경우, 양성자의 반감기는 1041 년 이하라고 생각되는 이론적 이유가 있습니다. [10] 중성자는 양성자의 반감기로 붕괴 할 것으로 예상된다.[10]
양성자가 전혀 붕괴하지 않는 경우, 별의 질량 물체는 여전히 사라지지만,더 천천히한다. 아래 참조 (양성자의 붕괴가 없는 미래).
이 타임 라인의 나머지는 양성자의 반감기가 약 1037 년이라고 가정한다.[10] 더 짧거나 또는 긴 양성자의 반감기는 과정을 가속하거나 감속 할 것이다. 이는 1037 년 후에, 모든 중입자 물질의 절반은 양성자 붕괴를 통해 감마선 광자와 렙톤으로 변환된다는 것을 의미한다.
모든 핵자 붕괴[편집]
- 1040 년
양성자의 추정 반감기를 감안할 때, 핵자는 우주가 1040 년에 약 1,000 반감기를 겪는 것이다. 이를 관점으로 풀어 쓰면, 현재 우주에는 약 1080개의 양자가 있다.[11] 이것은 핵자들이 1040 년을 겪는 동안 1000번 반으로 깎긴다는 얘기이다. 따라서 축퇴 시대가 끝날 때는 약½1,000 (약 10−301)개의 핵자가 남을 것이며, 따라서 우주에서는 핵자가 사라진다. 효율적으로 모든 중입자 물질은 광자와 렙톤으로 바뀔 것이다. 일부 모델은 1085 년에 관측 우주의 현재 직경보다 큰 직경을 가진 안정적인 포지트로늄 원자의 형성을 예상하고 이는 10141년 안에 감마선으로 차례로 붕괴하게 된다.[8] §IID, [12]
Black Hole Era[편집]
- 1040 years to 10100 years
After 1040 years, black holes will dominate the universe. They will slowly evaporate via Hawking radiation.[8], §IVG. A black hole with a mass of around 1 solar mass will vanish in around 2×1066 years. However, many of these are likely to merge with supermassive black holes at the center of their galaxies through processes described above long before this happens. As the lifetime of a black hole is proportional to the cube of its mass, more massive black holes take longer to decay. A supermassive black hole with a mass of 1011 (100 billion) solar masses will evaporate in around 2×1099 years.[13]
Hawking radiation has a thermal spectrum. During most of a black hole's lifetime, the radiation has a low temperature and is mainly in the form of massless particles such as photons and hypothetical gravitons. As the black hole's mass decreases, its temperature increases, becoming comparable to the Sun's by the time the black hole mass has decreased to 1019 kilograms. The hole then provides a temporary source of light during the general darkness of the Black Hole Era. During the last stages of its evaporation, a black hole will emit not only massless particles but also heavier particles such as electrons, positrons, protons and antiprotons.[3], pp. 148–150.
If protons do not decay as described above[편집]
In the event the proton does not decay as described above, the Degenerate Era will last longer, and will overlap the Black Hole Era. In a timescale of approximately 1065 years, apparently rigid objects such as rocks will be able to rearrange their atoms and molecules via quantum tunnelling, behaving as a liquid does, but more slowly.[14] However, the proton is still expected to decay, for example via processes involving virtual black holes, or other higher-order processes, with a half-life of under 10200 years.[8], §IVF For example, under the Standard Model, groups of 2 or more nucleons are theoretically unstable because chiral anomaly allows processes that change baryon number by a multiple of 3.
Dark Era and Photon Age[편집]
- From 10100 years and beyond
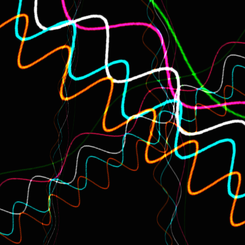
After all the black holes have evaporated (and after all the ordinary matter made of protons has disintegrated, if protons are unstable), the universe will be nearly empty. Photons, neutrinos, electrons, and positrons will fly from place to place, hardly ever encountering each other. Gravitationally, the universe will be dominated by dark matter, electrons, and positrons (not photons).[15]
By this era, with only very diffuse matter remaining, activity in the universe will have tailed off dramatically (compared with previous eras), with very low energy levels and very large time scales. Electrons and positrons drifting through space will encounter one another and occasionally form positronium atoms. These structures are unstable, however, and their constituent particles must eventually annihilate.[16] Other low-level annihilation events will also take place, albeit very slowly.
The universe now reaches an extremely low-energy state. What happens after this is speculative. It is possible that a Big Rip event may occur far off into the future. Also, the universe may enter a second inflationary epoch, or, assuming that the current vacuum state is a false vacuum, the vacuum may decay into a lower-energy state.[17] Finally, the universe may settle into this state forever, achieving true heat death. Presumably, extreme low-energy states imply that localized quantum events become major macroscopic phenomena rather than negligible microscopic events because the smallest perturbations make the biggest difference in this era, so there is no telling what may happen to space or time. It is perceived that the laws of "macro-physics" will break down, and the laws of "quantum-physics" will prevail.[18]
- ↑ 가 나 Adams & Laughlin (1997), §IIIC.
- ↑ The Future of the Universe, M. Richmond, lecture notes, "Physics 240", Rochester Institute of Technology. Accessed on line July 8, 2008.
- ↑ 가 나 다 라 마 The Five Ages of the Universe, Fred Adams and Greg Laughlin, New York: The Free Press, 1999, ISBN 0-684-85422-8.
- ↑ 인용 오류:
<ref>
태그가 잘못되었습니다;low_mass_lifetime
라는 이름을 가진 주석에 텍스트가 없습니다 - ↑ Adams & Laughlin (1997), §IIIF, Table I.
- ↑ p. 428, A deep focus on NGC 1883, A. L. Tadross, Bulletin of the Astronomical Society of India 33, #4 (December 2005), pp. 421–431, Bibcode: 2005BASI...33..421T.
- ↑ Reading notes, Liliya L. R. Williams, Astrophysics II: Galactic and Extragalactic Astronomy, University of Minnesota, accessed on line July 20, 2008.
- ↑ 가 나 다 라 인용 오류:
<ref>
태그가 잘못되었습니다;dying
라는 이름을 가진 주석에 텍스트가 없습니다 - ↑ G Senjanovic Proton decay and grand unification, Dec 2009
- ↑ 가 나 다 Adams & Laughlin (1997), §IVA.
- ↑ Solution, exercise 17, One Universe: At Home in the Cosmos, Neil de Grasse Tyson, Charles Tsun-Chu Liu, and Robert Irion, Washington, D.C.: Joseph Henry Press, 2000. ISBN 0-309-06488-0.
- ↑ 인용 오류:
<ref>
태그가 잘못되었습니다;dying-IIE
라는 이름을 가진 주석에 텍스트가 없습니다 - ↑ Particle emission rates from a black hole: Massless particles from an uncharged, nonrotating hole, Don N. Page, Physical Review D 13 (1976), pp. 198–206. doi 10.1103/PhysRevD.13.198. See in particular equation (27).
- ↑ 인용 오류:
<ref>
태그가 잘못되었습니다;twoe
라는 이름을 가진 주석에 텍스트가 없습니다 - ↑ Adams & Laughlin (1997), §VD.
- ↑ Adams & Laughlin (1997), §VF3.
- ↑ Adams & Laughlin (1997), §VE.
- ↑ 인용 오류:
<ref>
태그가 잘못되었습니다;dying-VID
라는 이름을 가진 주석에 텍스트가 없습니다